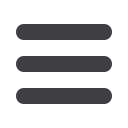
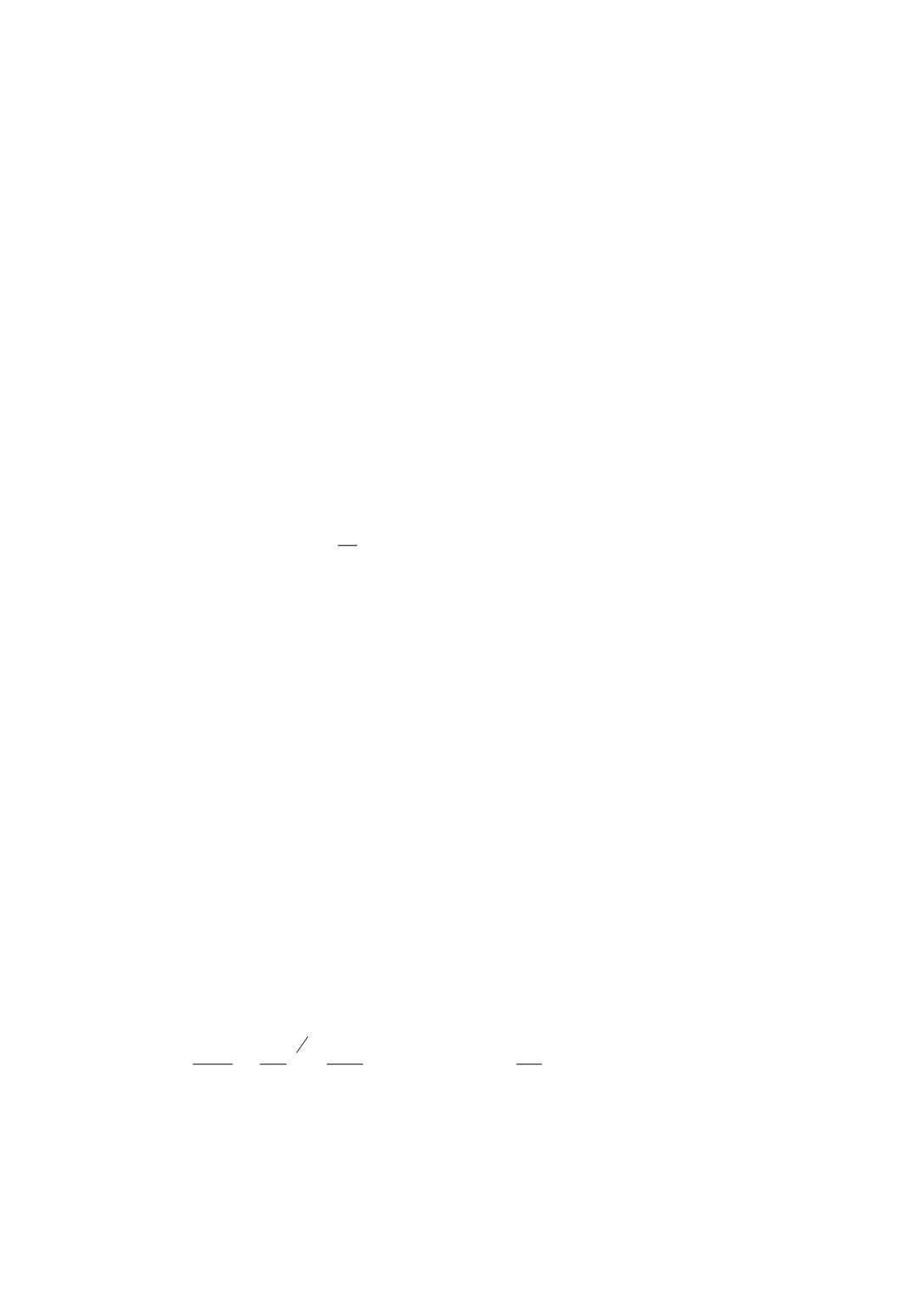
0
=
+ − −
recycle
reac
entr
in
m m mm
(4)
To describe the changes of the particle size distribution within the fluidized bed, mass
balances for each particle class
i
are also considered in the model according to Equation
5 by means of the particle mass fraction distribution
Q
3,i
. Here, the inlet particle size
distribution, the shrinking of the particles due to the chemical reaction as well as the
changes in the bed’s composition due to particle entrainment are considered.
0
,
,3
,
,3
,
,3
, ,3
=
Δ⋅
+
Δ⋅
− Δ⋅
− Δ⋅
i
recycle
recycle
i
reac
reac
i
entr
entr
i in
in
Q m Q m Q m Q m
(5)
Modeling the chemical reaction of the fluidized-bed reactor
In order to improve selectivity and yield, a lot of research work concerning the chemical
reaction was performed in the past, particularly on catalyst systems, on reaction
conditions or on the influence of the powders used. Despite the extensive research
activities, the reaction mechanism of the Mueller-Rochow reaction is still not fully
understood. Thus for modeling the chemical reaction, a reliable and robust reaction
constant approach is chosen, according to Equation 6.
RT
E
i
p
n
MeCl
i
iA
i
ek a
c c
,
−
⋅ ⋅
⋅
=
(6)
Here, the formation of the different products
i,
such as from Equation 1, depend on the
availability of the gaseous reactant chloromethane
c
MeCl
and on the availability of the
active centers on the particles surface that is assumed to correspond to the total surface
area of the silicon particles
a
p
. Both parameters are governed by the fluid dynamics of
the fluidized bed and so are connected to the fluid-dynamic submodel. The order of
reaction
n
i
, as well as the reaction constants
k
i
for each product component are
determined experimentally by means of the Mueller-Rochow pilot plant. In order to
describe the temperature dependency on the reaction constant, the Arrhenius approach
is used, whereas activation energies
E
A,i
were validated as well by means of the Mueller-
Rochow pilot plant. Furthermore,
R
represents the gas constant and
T
the corresponding
temperature of reaction.
Modeling the fluid dynamics of the fluidized-bed reactor
As the Equations 2-3 show, fluid-dynamic input data is required, such as the local
bubble fraction or the bubbles’ overall surface area for mass transport calculations.
These parameters are provided by a fluid-dynamic submodel, which describes the
bubble growth in the dense bed with height, by means of a volume-equivalent, spherical
bubble diameter,
d
v
, using Equations 7-10 according to Hilligardt and Werther [12].
Besides the bubble growth, the second term in Equation 7 takes the splitting of bubbles
on the basis of a mean bubble lifetime
Ȝ
into account, which becomes relevant
especially for Geldart A powders.
( )
b
v
b
v
u
d
dh
dd
λ
π
ε
3
9
2
3
1
−
¸
¹
·
¨
©
§
=
with:
g
u
mf
280
=
λ
(7)
The bubble volume fraction,
İ
b
which is also a height-dependent input variable for the
employed two phase model approach, is calculated from the visible bubble flow
V
լ
b
and
the bubble rise velocity
u
b
. Here,
g
denotes the acceleration due to gravity. The visible
162