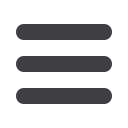
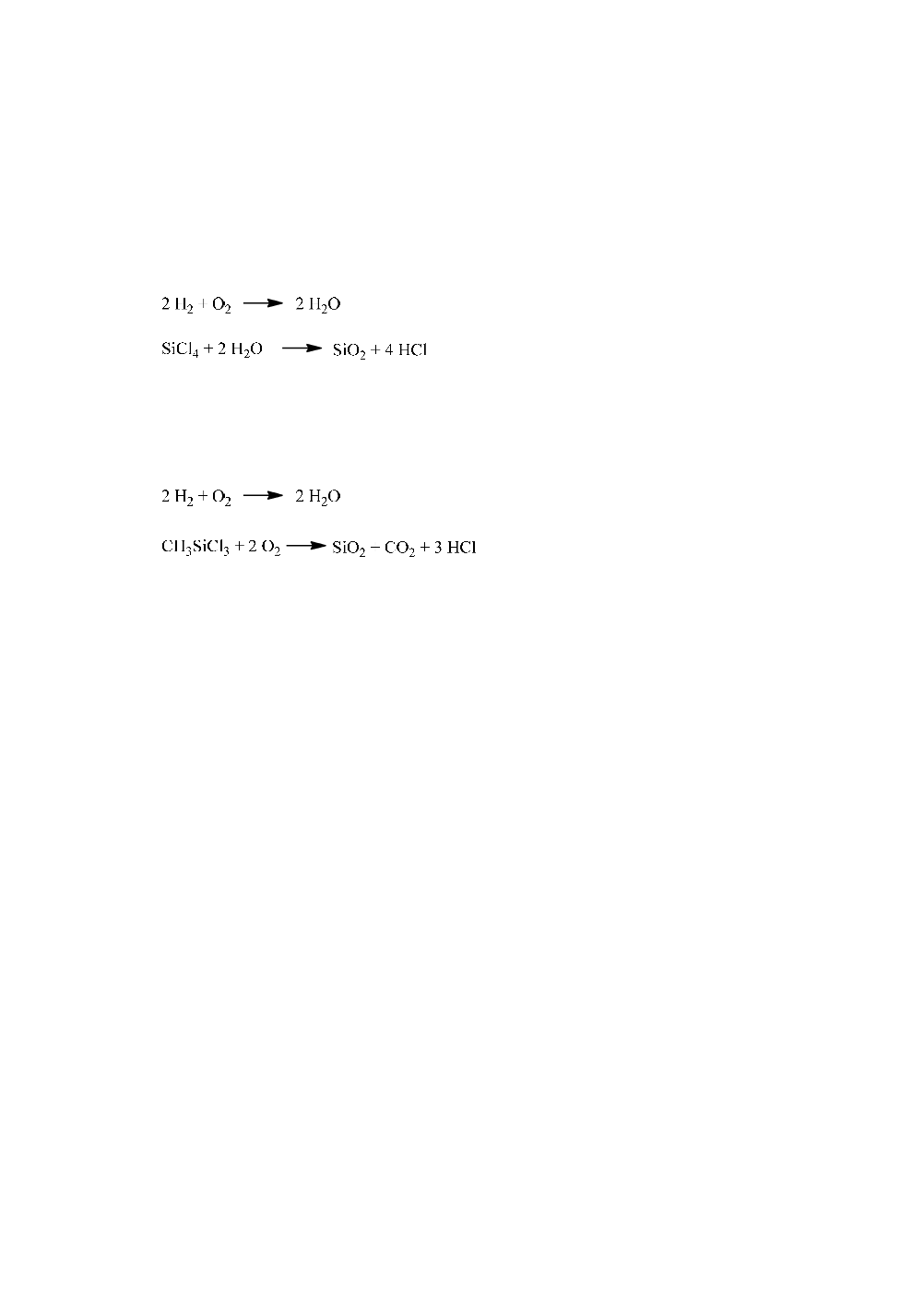
Target product of the Müller-Rochow methylchlorosilane process is
dimethyldichlorosilane, Me
2
SiCl
2
. However, an important side product is
methyltrichlorosilane, MeSiCl
3
, of 3 – 15% relative yield, which is also often used as a
raw material in pyrogenic silica production [2].
The formation of pyrogenic silica from SiCl
4
is a high temperature hydrolysis in a
hydrogen-oxygen flame according to Eqs. 1 and 2.
(1)
(2)
From thermodynamic data of Eq. 1 and 2, an adiabatic flame temperature of ca. 1700
K can be calculated.
The corresponding reaction of (CH
3
)SiCl
3
is a high temperature hydrolysis/oxidation
(Eqs. 3 and 4).
(3)
(4)
The oxidation of the methyl group results in a higher adiabatic flame temperature of
approximately1900 K. Based on the stoichiometry of eq. 4, no additional water is
needed for the hydrolysis of the Si-Cl bonds. However, the hydrogen-oxygen flame
reaction (eq. 3) is still needed to provide enough energy for the particle formation as
discussed below.
The particle formation is assumed to be a complex process of chemical reactions
combined with different particle growth processes. Fig. 1 schematically depicts this
process. Main parameters to control the particle growth are the absolute temperature
and the residence time of particles in a certain temperature range [3].
The notion is that in the very early stage of the process cascade SiO
2
molecules form,
which instantaneously fuse to so-called proto-particles of ca. 1nm in size (range 1 in
Fig. 1) [4]. This happens close to the hottest part of the flame. Due to the high
temperature, which is far above the melting temperature of amorphous SiO
2
(
ܶ
ௌைଶ
),
proto-particles are in fact droplets of liquid SiO
2
. Collision of proto-particles results in
particle growth by coalescence (s. range 2). Finally, primary particles of 5 – 50 nm in
diameter result. Their size primarily depends on temperature and residence time. The
viscosity of molten SiO
2
strongly depends on temperature and water content [5]. Hence,
with decreasing temperature the viscosity heavily increases and below a certain
temperature complete coalescence of particles upon collision is no longer possible. As
a consequence, particle-particle collision in this cooler part of the flame (range 3)
causes aggregation [6]. The primary particles within the aggregates are fused by sinter
bridges. When the temperature drops below the melting temperature of SiO
2,
agglomeration starts. Within the agglomerates the aggregates hold together due to
strong surface forces like London, Keesom, and van der Waals forces [3].
108