Role of silicon carbide (SiC) in silicon/ferro silicon (Si/FeSi) process
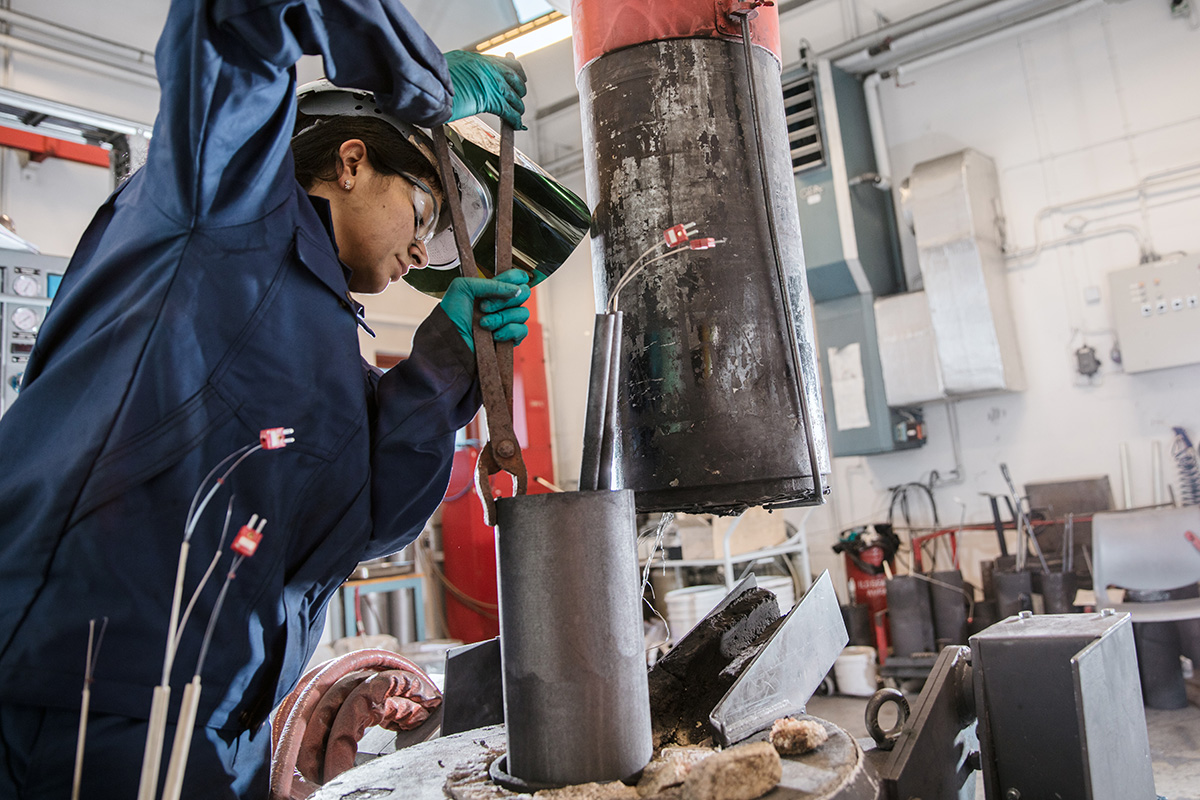
The demand for high quality silicon is rapidly increasing in the solar cell industry and for production of photovoltaic applications. To meet this demand, the industrial production of silicon must be made more effective. An extensive knowledge of the role of silicon carbide in the silicon production process is vital.
Silicon carbide – and its outstanding properties
Silicon carbide (SiC), commonly known as carborundum, is a unique compound of carbon (C) and silicon (Si) and plays a central part in the silicon production process. SiC is a non-oxide ceramic material with outstanding properties, which makes it an attractive material for many industrial applications.
Compared with other materials, SiC generally exhibits higher hardness and mechanical strength at higher temperatures, and has an excellent thermal conductivity. It also has a lower coefficient of thermal expansion, high melting point, high resistance to corrosion and oxidation, and a wide bandgap [2]. The excellent electronic properties of SiC make it a highly promising material in many industrial applications. SiC ceramics made from bio-carbon with anisotropic porosity is gaining more interest in the manufacturing of new lightweight, heat-resistant materials.
Silicon carbide- the important player in silicon production
SiC has two main roles in the industrial production of Si [3]:
-
- It acts as a requisite intermediate product for the final Si production.
- It plays a vital role in distributing the energy inside the Si furnace.
Extensive knowledge of SiC formation is essential for understanding the Si production.
Silicon is produced in submerged arc furnaces. Quartz (SiO2) and carbon materials such as charcoal, coal and petroleum coke are the raw materials used to produce silicon. During the Si production process, the carbon sources (carbon from the raw materials) are converted to SiC as a result of its interaction with silicon monoxide gas (SiO(g)) formed in the furnace. SiC exists in several forms without changing its composition known as its polytypes. The cubic modification of SiC is referred as β-SiC and the hexagonal modifications of SiC are collectively referred as α-SiC.
Si production process is a high temperature process. During the Si process the furnace temperature from the top to bottom ranges between 700 – 2000°C and above. The furnace body consists of shallow crucibles made of steel shells and furnace linings made of thick carbon blocks that can withstand extremely high temperatures. The process requires a lot of electrical energy, which is delivered through the three consumable carbon electrodes located at the center of the furnace. The principal parts of a modern Si plant is as shown in Figure 1.
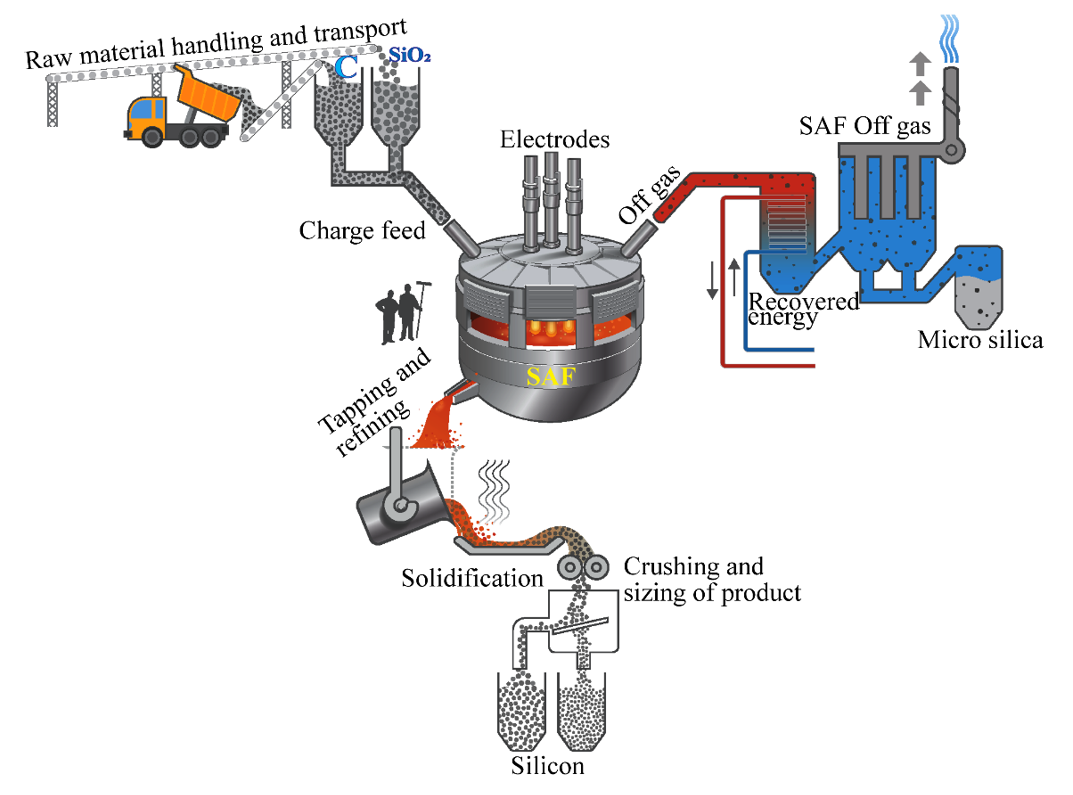
Uncovering the secrets of the furnace; SiC deposits observed during furnace excavations
Though the properties of commercial SiC are well documented, the formation processes as well as properties of the SiC formed during the industrial production of Si are not investigated to the same extent. This has been a major motive for my research. Through my work, I have analyzed formation of different types of SiC from different carbon sources (charcoal, coal and petroleum-coke) predominantly used in the Si production process.
We carried out furnace excavations when industrial Si production furnaces were shut down (due to problems with operation or for furnace maintenance). After excavations, we analyzed the samples from different locations in the furnace, at the macroscopic and microscopic levels using different types of electron microscopes. These investigations resulted in a detailed and better understanding of the chemical reactions and material flows inside the furnace.
Our furnace excavations and investigations revealed:
- accumulation of SiC in the form of crusts.
- large gas channels in the SiC crust. Inside the gas channels, precipitates of SiC crystals in the form of dendrites were also noticed.
- the structure of SiC crust (α-SiC) that was observed after the excavations were entirely different from the SiC that had directly formed from the carbon materials (β-SiC) reacting with SiO(g).
Based on our results, we could develop a new conceptual model for the processes going on inside the furnace during Silicon production. Our work could help to adjust the production processes further, so they become more effective and produce higher quality silicon. Though the physical and structural properties of commercial SiC are well documented, the formation processes as well as properties of SiC formed during the industrial production of Si have not been investigated extensively. This motivated a part of research presented in this thesis, where I predominantly analyzed formation of different types of SiC from various carbon sources (charcoal, coal and petroleum-coke) that are generally used in the industrial production of Si. Analyzing the characteristics of SiC produced from different carbon materials and the changes they undergo at higher temperatures are relevant to the Si industry and several other fields, including development of high-temperature power electronic devices.

Outcome from my research
In the industrial Si production process, a temperature gradient exists in the furnace from its top to the bottom part ranging from 500 to 2000 °C and above. Several types of carbon materials are used to produce SiC in the Si process. A number of aspects are evaluated in choosing the type of carbon material to be used industrially, such as its strength and SiO-reactivity. As a basis for future investigations of the various SiC producing mechanisms, I have investigated the structure of the SiC formed from different carbon materials at temperatures ranging from 1674 to 2450 °C and compared them with the industrial SiC samples. We documented the extent of α-SiC formation from β-SiC, based on the original type of carbon materials. Our work examined whether the presence of elemental Si in the β-SiC affects the extent of transformation to α-SiC.
- β-SiC produced from different carbon materials were heat treated to a temperature ranging from 2100-2450 °C. The structure of SiC and factors influencing the transformation to high temperature SiC (α-SiC) were studied and published [6]. This study validates the efficacy of charcoal-converted β-SiC particles to easily transform to α-SiC at 2100 °C, even without a significant presence of any known impurities in the original carbon source. A pivotal finding from this study was the presence of elemental Si in β-SiC particles, which played a crucial role in enhancing the extent of their transformation to α-SiC.
- We believed that Si is produced at a temperature of 1811 °C and a SiO(g) pressure of 0.67 bar. Interestingly, in this study, formation of elemental Si in SiC particles was observed even at 1674 °C at higher SiO pressures. The formation of Si in SiC particles was found to be directly proportional to the increasing temperatures. Thus, the current findings prove that it is possible to produce Si in SiC particles quite higher up in the furnace, even at temperatures lower than 1800 °C, provided the partial pressure of SiO(g) is favorable.
- Being one of the main electrical conducting materials in the crater wall, SiC plays an active role in the energy distribution inside the furnace for Si process. Despite having same composition, each SiC polytypehas its own unique set of electronic properties. Conductivity of SiC varies with temperature, depending on the number of polytypes present in it. This study identified and analyzed the structures of SiC and the various polytypes formed in them, produced over a wide range of temperature (1647–2450 °C) attainable in laboratory-scale experiments. Results obtained from our study would certainly provide the industry with an insight into the key factors influencing Si production and intermediate formation of SiC in the process, and the importance of choosing the right carbon material, for efficiently producing Si.
- Our microscopic analyses discovered SiC crystals in the shape of micro-bullets grown on the charcoal substrate. Those findings were published [5] and they provide the SiC ceramic industry an opportunity to explore the possibility of developing novel light-weight high-temperature resistant materials from bio-carbons that possess
Electronic version of my PhD thesis for more details.
References
[1] A. L. Ortiz, F. Sánchez-Bajo, F. L. Cumbrera, and F. Guiberteau. The prolific polytypism of silicon carbide. Journal of Applied Crystallography, 46(1): 242–247, 2013.
[2] G. L. Harris. Properties of silicon carbide. INSPEC, the institution of Electrical Engineers, United Kingdom, 1995.
[3] A. Schei, J. K. Tuset, and H. Tveit. Production of high silicon alloys. Tapir Trondheim, Norway, 1998.
[4] M. Tangstad, M. Ksiazek, and J. E. Andersen. Zones and materials in the Si furnace. In Proceedings: Silicon for the chemical and solar industry XII, Trondheim, Norway, June, pages 24–27, 2014.
[5] S. Jayakumari, P. E. Vullum, A. T. J. van Helvoort, and M. Tangstad. SiC crystalline micro bullets on bio-carbon based charcoal substrate. Journal of Crystal Growth, 545, 2020.
[6] S. Jayakumari and M. Tangstad. Transformation of β-SiC from Charcoal, Coal, and Petroleum Coke to α-SiC at Higher Temperatures. Metallurgical and Materials Transaction B, 2020.
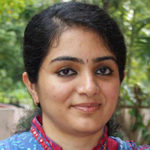
Sethulakshmy Jayakumari
Sethulakshmy Jayakumari is a researcher at the Department of Materials Science and Engineering. She primarily works at the Metal Production SFI centre.
Tags In
Search
Søk
Categories
- Arctic Research
- Arkitektur
- Bærekraft
- Bioingeniørfag
- Biologi
- Biology
- Biomedical Laboratory Science
- Biotechnology
- Bioteknologi
- Chemical Engineering
- Chemistry
- Climate
- Computer Science
- Datateknologi
- Digital
- Elektronikk
- Energi
- Energi
- Energy
- Engineering
- Engineering
- Environment
- Food Science
- Forskning
- Fysikk
- Fysikk
- Havbruk
- Informasjonsteknologi
- Informasjonsteknologi
- Ingeniørvitenskap
- Kjemi
- Kjemisk prosessteknologi
- Kjemisk prosessteknologi
- Kreftbehandling
- Kybernetikk
- Marine Technology
- Materialer
- Materials Science
- Materialteknologi
- Matvitenskap
- Meninger
- Miljø
- Min ph.d.
- My PhD
- My PhD
- My postdoc
- Nanotechnology
- Nanoteknologi
- Ocean
- Oil and gas
- Physics
- Research
- Simulering og visualisering
- Spør en forsker
- Studentliv
- Sustainability
- Ukategorisert
- Universitetsliv
- University Life
Kategorier
- Arctic Research
- Arkitektur
- Bærekraft
- Bioingeniørfag
- Biologi
- Biology
- Biomedical Laboratory Science
- Biotechnology
- Bioteknologi
- Chemical Engineering
- Chemistry
- Climate
- Computer Science
- Datateknologi
- Digital
- Elektronikk
- Energi
- Energi
- Energy
- Engineering
- Engineering
- Environment
- Food Science
- Forskning
- Fysikk
- Fysikk
- Havbruk
- Informasjonsteknologi
- Informasjonsteknologi
- Ingeniørvitenskap
- Kjemi
- Kjemisk prosessteknologi
- Kjemisk prosessteknologi
- Kreftbehandling
- Kybernetikk
- Marine Technology
- Materialer
- Materials Science
- Materialteknologi
- Matvitenskap
- Meninger
- Miljø
- Min ph.d.
- My PhD
- My PhD
- My postdoc
- Nanotechnology
- Nanoteknologi
- Ocean
- Oil and gas
- Physics
- Research
- Simulering og visualisering
- Spør en forsker
- Studentliv
- Sustainability
- Ukategorisert
- Universitetsliv
- University Life